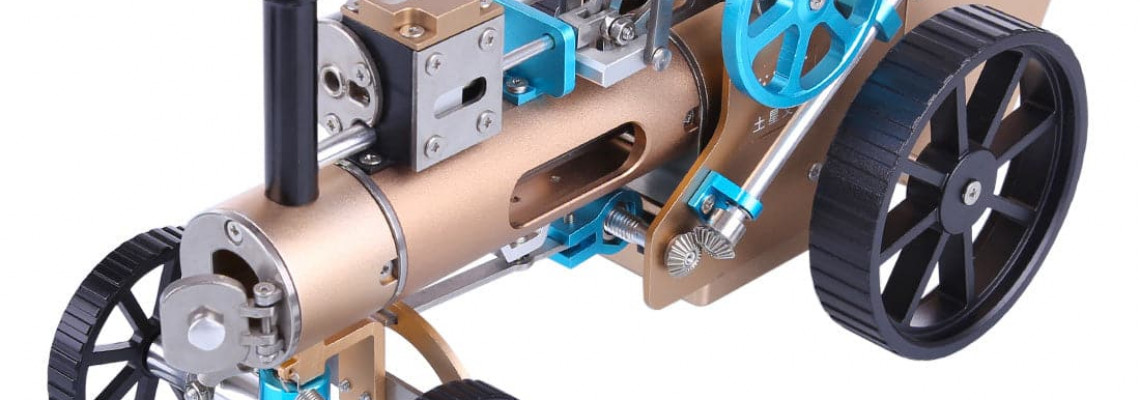
10 hp Steam Engine for Sale
10 horsepower Steam Engine for Sale For more than a century, steam turbines and engines have been the foundation of power generation. The design, functionality, and use of these devices have changed dramatically from the early stages of industrialization to the present. A steam turbine is a machine that transforms steam's thermal energy into mechanical work that can power mechanical operations or produce electricity.
Turbine Machine: Energy conversion is the fundamental idea underlying a turbine machine. In a steam turbine, high-pressure, high-temperature steam is transformed into rotational energy through a system of blades or vanes. The rotating energy then powers a generator to create electricity.
Working premise of a Turbine Generator: A turbine generator operates on a simple but intricate premise. The turbine's blades rotate when steam flows through it. The generator's rotor is driven by this spinning motion, which uses electromagnetic induction to create electricity. The turbine's design, the steam's temperature and pressure, and the generator's quality are some of the variables that affect how efficient this operation is.
Types of Steam Turbines
Steam turbines come in various types, each designed for specific applications and operating conditions. The two primary categories are impulse and reaction turbines.
Definition and Function of an impulse turbine: An impulse turbine works by forcing steam onto the turbine blades at high speed through nozzles. Potential energy is transformed into kinetic energy as the steam pressure drops across the nozzles. One popular example of an impulse turbine is the Curtis stage turbine, which uses several stages to extract energy more effectively.
Curtis Stage Turbine: Named for its creator, Charles G. Curtis, this turbine maximizes efficiency by progressively lowering the energy of the steam through a sequence of stationary and spinning blades. In situations when a considerable drop in steam pressure is necessary, the Curtis stage turbine works very well.
Impulse Reaction Turbine: Unlike impulse turbines, reaction turbines work by expanding and accelerating steam as it flows through stationary and moving blades—differences in design result in differences in optimal operating conditions, maintenance needs, and efficiency.
Steam Cycle and Its Components
The basic thermodynamic mechanism that drives the operation of steam turbines in power generation is known as the steam cycle or Rankine cycle. Optimizing the performance and efficiency of thermal power plants requires a thorough understanding of the steam cycle and its constituent parts. The complexities of the steam cycle diagram, the operation of steam condensers, and the role of steam nozzle converts in turbine energy conversion are covered in detail in this section.
The Steam Cycle Diagram Explained
Fundamentally, the steam cycle diagram shows the successive steps that steam takes to produce electrical and mechanical energy. Heating, expansion, condensation, and pumping are the main phases. Here is a detailed explanation:
Water Pumping (Pumping Stage):
Function: Water is fed into a high-pressure boiler from a low-pressure reservoir to start the cycle.
Procedure: The pump raises the water's pressure to prepare it for effective heating. Pumps are essential to preserving the cycle's continuity even though they use energy.
Boiling (Heating Stage):
Function: The boiler heats high-pressure water to create steam.
Process: The water's temperature and pressure are raised until it produces saturated or superheated steam by applying heat, usually from the burning of fossil fuels or other energy sources.
Expansion in the Turbine (Expansion Stage):
Function: Thermal energy is transformed into mechanical work as the high-pressure steam expands through the turbine.
Process: Steam gains velocity and loses temperature and pressure as it moves through the turbine blades, which propels the turbine rotor to produce rotational energy.
Condensation (Condensation Stage):
Function: The steam is cooled and condensed back into water in the condenser after expanding.
Procedure: The condenser uses cooling water from a nearby source to remove any remaining heat from the steam. The turbine's efficiency and vacuum maintenance depend on this phase transition from gas to liquid.
Recirculation (Return to Pumping Stage):
Function: The cycle is finished when the condensed water is put back into the pump.
Process: The system maintains the power generation process by recycling water, guaranteeing a steady flow of the working fluid.
The steam cycle diagram graphically shows these phases and emphasizes energy flow and the conversion of water into steam and vice versa. The boiler, turbine, condenser, and pump—all connected by lines showing the flow of water and steam—are usually the main features on the diagram.
Role of Steam Condensers
An essential component of the steam cycle's effectiveness is steam condensers. Their main job is to turn the turbine's exhaust steam back into liquid water so that the cycle can continue. Several important factors highlight the significance of condensers:
Heat Rejection:
Function: Following its passage through the turbine, the steam's leftover heat is rejected via condensers.
Process: Condensers eliminate heat to maintain a low-pressure environment at the turbine's exhaust, which is crucial for optimizing the turbine's efficiency.
Maintaining Vacuum:
Function: The back pressure on the turbine is decreased by creating a vacuum in the condenser.
Procedure: By drawing more energy from the steam during expansion, a lower exhaust pressure enables the turbine to operate more efficiently.
Water Recovery:
Function: Condensers facilitate water recovery for cycle reuse.
Process: Condensers minimize water usage and operating expenses by converting the steam back into water, ensuring that the working fluid stays in the system.
Types of Condensers:
Surface Condensers: Cooling water absorbs heat from the steam without coming into direct contact with it.
Jet condensers: Allow for quick heat transfer by directly introducing a spray of cooling water into the steam.
Air-Cooled Condensers: These units use the surrounding air to chill and condense the steam, which lessens the need for water.
A steam condenser's efficiency has a direct effect on the power plant's overall efficiency. Advanced materials and design advancements continuously improve condenser performance, helping to produce power more economically and ecologically.
Steam Nozzle Converts: Energy Conversion in Turbines
The precise transformation of thermal energy into kinetic energy is accomplished by steam nozzle converters, which are essential parts of steam turbines. Their functionality and design have a big impact on the turbine's performance and efficiency. Here's a detailed look at how they work:
Function of Steam Nozzles:
Energy Conversion: Steam nozzles propel the steam to high speeds, which provides the ideal amount of force to the turbine blades.
Pressure Reduction: Steam's pressure decreases as it moves through the nozzles, making it easier to transform pressure energy into kinetic energy.
Types of Steam Nozzles:
Convergent Nozzles: Ideal for subsonic steam speeds, these nozzles are narrow toward the exit.
Convergent-Divergent Nozzles: These nozzles provide supersonic steam velocities, which are essential for high-efficiency turbines, by narrowing at the throat and widening near the exit.
Design Considerations:
Shape and Geometry: The nozzle's shape must minimize energy losses and turbulence while ensuring smooth steam acceleration.
Material Selection: To survive the severe conditions inside the turbine, materials that can sustain high temperatures are crucial.
Operational Impact:
Blade Loading: By distributing steam evenly throughout turbine blades, well-designed nozzles reduce mechanical stress and uneven loading.
Efficiency Optimization: Effective steam nozzles raise the turbine's overall efficiency by maximizing the transformation of thermal energy into mechanical work.
Advanced Technologies:
Variable Geometry Nozzles: These offer flexibility and maintain peak performance under a range of loads by enabling nozzle size and shape modifications based on operating conditions.
Aerodynamic Improvements: Including aerodynamic elements improves steam flow characteristics and lowers energy losses.
Thus, steam nozzle converters are essential to ensure that the turbine efficiently captures the energy contained in the steam. Nozzle design advancements keep increasing turbine efficiency, making energy generation systems more robust and sustainable.
Integration of Components in the Steam Cycle
Maintaining a steady and effective power generation process requires the smooth integration of the boiler, turbine, condenser, and pump that make up the steam cycle. Every component responds to variations in operating circumstances and load demands through dynamic interaction.
Thermodynamic Efficiency:
The temperature and pressure differential between the boiler and condenser affect the steam cycle's efficiency.
Maximizing the boiler's temperature and pressure while lowering the condenser pressure increases the cycle's efficiency.
Heat Exchange Optimization:
Energy use is optimized by efficient heat exchange in the boiler and condenser, which lowers waste and boosts efficiency.
Sophisticated heat exchanger designs, such as superheaters and economizers, further improve thermal efficiency.
Control Systems:
Automated control systems regulate the flow of water, steam, and cooling agents to maintain ideal working conditions.
Expansion in the Turbine (Expansion Stage):
Function: After entering the turbine, the high-pressure steam expands and releases pressure. The turbine blades are driven by this expansion, which transforms the thermal energy of the steam into mechanical work.
Process: As the steam moves through several turbine stages, each intended to extract as much energy as possible, its temperature and pressure drastically decrease. Electricity is then produced by an electrical generator powered by the mechanical labour the turbine produces.
Condensation (Condensation Stage):
Function: The low-pressure steam travels through a turbine before cooling and turning back into water in a condenser.
Procedure: After releasing its residual thermal energy, the steam condenses into water in the condenser. Usually, cooling water from a nearby lake, river, or cooling tower helps in this process. Because it enables the water to be recycled back into the boiler, the condensation process is essential to preserving the steam cycle's efficiency.
Feedwater Heating (Optional):
Function: The condensed water may go through a number of feedwater heaters before returning to the boiler.
Procedure: To preheat the water, feedwater heaters employ steam collected from different turbine stages. This lowers the quantity of fuel needed to bring the water to a boil, increasing the cycle's overall efficiency.
Role of Steam Condensers
Because they make it easier for steam to condense back into water, steam condensers are essential to the steam cycle's effectiveness. Among their main duties are:
Maintaining Vacuum Pressure: Condensers lower the back pressure on the turbine by condensing steam and producing a vacuum, which enables the turbine to run more effectively.
Heat Rejection: Condensers extract waste heat from the cycle and usually move it to air or water for cooling.
Water Recovery: Condensers allow water to be reused in the cycle by turning steam back into water, which minimizes the impact on the environment and the need for fresh water.
Surface condensers and evaporative condensers are two examples of the various types of condensers appropriate for multiple plant designs and operating environments. The selection of the condenser type impacts the power plant's overall efficiency and maintenance needs.
Steam Nozzle Converts: Energy Conversion in Turbines
Steam nozzles are essential parts of turbines' energy conversion process. The turbine blades are propelled by the kinetic energy they produce from the thermal energy of steam. The following are the main uses and varieties of steam nozzles:
Energy Conversion: Steam is propelled to high speeds by steam nozzles, which transform its thermal energy into kinetic energy. The turbine blades then revolve as a result of the high-velocity steam's impact.
Types of Nozzles:
Convergent Nozzles: These nozzles, usually found in impulse turbines, are employed when the steam flow needs to be accelerated.
Divergent Nozzles: Less frequently found in steam turbines, these nozzles are used when the steam flow needs to be reduced.
Convergent-Divergent Nozzles: Frequently employed in high-pressure settings, these nozzles first converge to speed up steam before diverging to control pressure drops.
The efficiency and design of the steam nozzles greatly impact the turbine's total performance, which affects both power production and operational efficiency.
Low-Pressure Turbines in Thermal Power Plants
Low-pressure turbines (LPTs) are essential parts of thermal power plants, particularly in the later phases of the steam cycle, when the steam pressure greatly decreases after passing through the high-pressure and intermediate-pressure turbines. This section examines the Function of LPTs, design factors, and their relationship to intermediate-pressure (IP) steam.
Role and Importance of Low-Pressure Turbines
Steam that has already expanded via high-pressure (HP) and intermediate-pressure (IP) turbines is handled by low-pressure turbines. Among the primary duties of LPTs are:
Energy Extraction: Steam has a substantial amount of energy even at low pressure. LPTs extract the residual energy in the steam and transform it into mechanical work that can power a generator.
Operational Efficiency: By optimizing the energy recovered from steam prior to its condensing in the condenser, LPTs help the power plant operate more efficiently overall. They are made to function well at low temperatures and pressures, frequently handling steam that is just marginally above saturation.
Design and Efficiency Considerations
Low-pressure turbine design presents a number of particular difficulties.
Blade Design: Compared to HP and IP turbine blades, LPT blades are usually longer and have a bigger diameter. This design makes effective energy extraction from low-pressure steam possible. Nevertheless, the larger blades also result in mechanical loads, which makes aerodynamic design and material choice crucial.
Moisture Handling: Steam frequently achieves saturation as it expands in the LPT, which causes moisture to form. If this moisture is not adequately controlled, it may erode the turbine blades. As a result, LPTs are made with characteristics that reduce damage from moisture.
Thermal Expansion: Because of their size and operational environment, LPTs require strict control of thermal expansion. The design of turbine casings and blades must allow for expansion without resulting in misalignment or mechanical failure.
Interaction with IP Steam Pressure
Intermediate-pressure turbines and low-pressure turbines work together in a coordinated fashion:
Steam Routing: The steam is routed into the LPT after going via the IP turbine. At this stage, the steam's temperature and pressure are crucial for maximizing the LPT's performance. To guarantee that the LPT functions within the constraints of its design, IP steam pressure needs to be carefully controlled.
Efficiency Optimization: Power plant operators can maximize the performance of both the IP and LPT stages by carefully regulating the IP steam pressure and temperature. This optimization frequently entails controlling the steam flow rate between the turbine stages and optimizing the reheating procedure.
Control and Regulation in Steam Turbines
In addition to their architecture, steam turbines require complex control and regulatory systems to operate efficiently. Under various load scenarios, these mechanisms guarantee the turbine's safe, dependable, and effective operation. This section covers the Function of steam governors, control systems, and safety features.
What is a Steam Governor?
A steam governor is a device that controls the flow of steam into a steam turbine, hence regulating its speed and output. A steam governor's primary duties include:
Speed Regulation: Regardless of variations in load demand, the governor modifies the steam flow to maintain a steady turbine speed. Differences in turbine speed can cause frequency changes in the electrical grid, which is essential for constant power generation.
Load Matching: The governor controls the steam flow to ensure that the turbine's power output corresponds to the load requirement. This keeps the grid stable and prevents the turbine from being overloaded.
Safety Control: Governors also act as safety measures by keeping the turbine from exceeding its speed limit. To prevent mechanical damage to the turbine in the event of an abrupt drop in load, the governor rapidly lowers the steam flow.
Steam Turbine Control Mechanisms
In order to guarantee effective and secure operation, steam turbine control systems are intricate and comprise a number of interconnected components. Turbine control systems' essential parts include:
Throttle Values: Throttle valves control the steam flow into the turbine. The control system manages the quantity of steam entering the turbine by varying the throttle valves' opening, which in turn controls the turbine's speed and power output.
Extraction Control: In certain turbines, steam is drawn out in phases for purposes such as heating. By controlling the quantity of steam that is diverted from the turbine, the extraction control system strikes a balance between power generation and other operational requirements.
Reheat Control: The control system in power plants with reheat cycles regulates the reheating process, making sure that the steam that re-enters the turbine at intermediate stages is at the ideal pressure and temperature.
Emergency Stop Systems: The turbine control system has features to quickly shut down the turbine in the event of an emergency or malfunction. Swiftly halting the turbine may entail closing throttle valves and applying brakes.
Safety Features and Efficiency Improvements
Contemporary steam turbines come with a number of safety features and efficiency improvements, such as:
Overspeed Protection: Turbines are equipped with sensors and automated shutdown systems that kick in if the turbine surpasses its maximum safe speed in order to guard against damage from overspeeding.
Vibration Monitoring: Monitoring turbine vibrations can identify imbalances or mechanical issues early on, enabling prompt maintenance and averting catastrophic failures.
Load-Shedding Capability: To safeguard both the turbine and the electrical system, turbines can be built to swiftly lower load or shut down in the event of grid disruption.
Advanced Control Algorithms: Modern control systems employ complex algorithms to maximize turbine operation and increase efficiency by adjusting the steam flow, temperature, and pressure in real time.
Modern Steam Engines and Small-scale Applications
Although the power generating sector is dominated by large-scale steam turbines, modern steam engines are nevertheless used in specialized, smaller-scale settings. This section examines the characteristics, benefits, and uses of small-scale steam engines, including those currently on the market.
10 hp Steam Engine for Sale: Applications and Advantages
A 10-hp steam engine is a compact, adaptable power source that may be utilized in a variety of situations, especially off-grid or in areas without easy access to electricity. Important attributes and advantages consist of the following:
Portability: These engines are perfect for off-grid applications such as sawmills and agricultural enterprises because they are small and portable.
Fuel Flexibility: In regions with limited resources, small steam engines may operate on a range of fuels, such as wood, coal, and biomass.
Reliability: Steam engines are resilient and require less maintenance than internal combustion engines because they have fewer moving parts. This makes them appropriate for long-term, unsupervised operation.
Educational Use: Thermodynamics and mechanical engineering concepts are also illustrated using these engines in academic contexts.
Modern Steam Engines for Sale: Advances in Design and Efficiency
Many technological developments have been introduced into contemporary steam engines, which are now for sale, to increase their efficiency and lessen their negative effects on the environment. Among these developments are:
Better Materials: The materials used in modern steam engines are more sophisticated and durable because they can tolerate higher temperatures and pressures.
Compact Designs: These engines are now easy to install and run thanks to engineering advancements that have produced lighter and more compact designs.
Improved Control Systems: Modern steam engines frequently include digital control systems that optimize fuel use, track engine performance, and lower pollutants.
Renewable Fuel Options: In keeping with environmental objectives, several contemporary steam engines are built to run on renewable fuels like wood chips or other biomass.
These engines are employed in specialized fields such as marine propulsion, small-scale power generation, and projects for hobbyists or educational purposes.
High-Power and Industrial Applications
Steam turbines are also essential in high-power and industrial applications, where their capacity to produce significant amounts of energy effectively is highly prized. This section examines the application of 50kW steam turbines and turbine propellers in industrial settings.
50kW Steam Turbine: Use Cases and Performance
A mid-sized power generation device appropriate for a range of industrial uses is a 50kW steam turbine. Important use cases consist of:
Industrial Process Heat: Steam is needed in many industries, including the production of chemicals and food, to generate electricity and provide process heat. These procedures can incorporate a 50kW steam turbine, which produces steam for heating as well as electricity.
Combined Heat and Power (CHP) Systems: These systems greatly increase total energy efficiency by using steam turbines to produce electricity and capture waste heat to utilize for heating.
Off-Grid Power Generation: When combined with a biomass boiler or other renewable energy sources, a 50kW steam turbine can offer a dependable power source for isolated industrial locations.
Integration in Industrial Power Generation
Several important factors need to be taken into account when integrating steam turbines into industrial power generation:
Fuel Source: The turbine's design and functionality are impacted by the fuel selection, which can include natural gas, coal, or biomass. Because they are sustainable and have a lower carbon footprint, biomass-fueled turbines are becoming increasingly popular.
Load matching: Industrial sites frequently have different power requirements. Steam turbines often need complex control systems to adapt their output effectively to these variations.
Environmental Impact: The design of contemporary steam turbines takes the environment into account. This entails cutting emissions, increasing fuel economy, and utilizing carbon capture and storage (CCS) and other technologies.
Wood Gasification and Wood-burning Engines
Wood gasification and wood-burning engines are sustainable methods of producing electricity, especially in regions with a wealth of biomass resources. This section covers the utilization of wood gas electric generators, wood-burning engine technology, and the wood gasification process.
The Wood Gasification Process: From Biomass to Energy
Pyrolysis: When wood is burned without oxygen, it breaks down into volatile gasses and charcoal. A mixture of hydrogen, carbon monoxide, methane, and other hydrocarbons is produced at this stage.
Combustion: To supply the heat required for pyrolysis and the ensuing processes, a tiny amount of the biomass is burned in a regulated setting.
Reduction: When the volatile gases generated during the pyrolysis step react with a bed of hot charcoal, a cleaner gas mixture is created. Carbon monoxide, hydrogen, and trace amounts of methane make up the majority of this mixture.
Gas Cleaning: After the production gas has been cleaned of tar, particles, and other contaminants, it can be used directly in boilers, turbines, and internal combustion engines.
The wood gasification process is incredibly effective because it can transform a sizable amount of biomass's energy content into usable gas. This gas can be utilized as a chemical feedstock or to produce heat or energy.
Wood-burning Engine Technology and Applications
The producer gas produced by wood gasification is what wood-burning engines, commonly referred to as wood gas engines, are made to run on. Important attributes and uses consist of:
Engine Design: These engines are usually modified internal combustion engines that can burn a combination of air and gas. They are frequently utilized in off-grid or rural places where wood is easily accessible for fuel.
Applications: Power generation, agricultural equipment, and backup generators are just a few of the uses for wood-burning engines. In places where traditional fuels are either unavailable or prohibitively expensive, they provide a renewable energy option.
Benefits: Using wood as fuel is carbon-neutral, which means that the carbon absorbed by the trees during their growth balances the carbon dioxide generated during combustion. Because of this, wood-burning engines are a green choice.
Wood Gas Electric Generator: A Sustainable Power Source
The producer gas from wood gasification is transformed into power by wood gas electric generators. In decentralized power generation systems where fuel availability and sustainability are important factors, these generators are especially helpful. Important elements consist of:
Generator Efficiency: The quality of the producer gas and the generator's design determine how efficient wood gas electric generators are. Contemporary designs are more dependable and efficient, which makes them appropriate for prolonged use.
Renewable Energy: Wood gas electric generators provide a sustainable and renewable energy source by using biomass as fuel, which lessens dependency on fossil fuels and greenhouse gas emissions.
Community Power Solutions: Wood gas generators can offer a dependable source of electricity in isolated or rural locations, boosting local economies and raising standards of living.
Self-sustaining Power Systems
Self-sustaining power systems are made to function autonomously, producing and preserving energy without the assistance of outside power sources. This section explores the advancements in renewable energy technology that enable self-sustaining power generation systems.
Understanding Self-sustaining Power Generators
With little external input, a self-sustaining power generator is a system that constantly produces its energy, frequently from renewable resources. Important features include:
Renewable Energy Sources: These generators usually use renewable energy sources like solar, wind, hydro, or biomass to run continuously.
Energy Storage: Self-sustaining systems frequently incorporate energy storage devices, such as batteries or flywheels, to store extra energy generated during high generation for use during low generation.
Automation and Control: State-of-the-art control systems monitor self-sustaining generator performance, maximizing their efficiency and guaranteeing that they react appropriately to variations in resource availability or energy demand.
Innovations in Renewable Energy Technologies
The efficiency and viability of self-sustaining power systems have increased due to recent developments in renewable energy technologies. Examples of innovations are:
Better Solar Panels: New designs and materials have made solar panels more efficient, enabling them to gather more energy from the same amount of sunlight.
Developments in Wind Turbines: Wind power is now more accessible and dependable due to the increased efficiency of modern wind turbines, which can produce electricity at lower wind speeds.
Energy Storage Advancements: Due to advancements in battery technology, such as solid-state batteries, which have increased energy storage capacity and efficiency, longer durations of self-sustaining operation are now possible.
Hybrid Systems: By integrating several renewable energy sources, such as wind and solar, into one system, dependability is increased, and power generation is guaranteed to continue even if one resource is unavailable.
The energy of the future lies in self-sustaining power systems, which provide a viable option for remote industrial operations, off-grid communities, and places with erratic grid connectivity.
Turbine Generators: Working Principle and Operation
Turbine generators, which transform turbines' mechanical energy into electrical energy, are crucial parts of power generation systems. This section examines the integration of turbines and generators in power plants, addresses efficiency problems, and describes the basic operation of turbine generators.
How Turbine Generators Work
The fundamental idea behind a turbine generator is the use of electromagnetic induction to transform mechanical energy from a turbine into electrical energy. The procedure consists of:
Mechanical Energy Input: The turbine, powered by steam, water, or wind, rotates a shaft attached to the generator.
Electromagnetic Induction: The generator's revolving shaft turns a rotor encircled by a magnetic field. As the rotor moves inside this magnetic field, electricity is produced, causing an electric current to flow through the stator windings.
Power Output: The electrical energy produced is either used to directly power equipment or sent to the power grid. The turbine's speed, the magnetic field's intensity, and the generator's efficiency all affect the amount of power produced.
Efficiency and Power Output Considerations
Numerous things affect a turbine generator's efficiency:
Turbine Efficiency: The turbine's efficiency is a major factor influencing the generator's total efficiency. Variables like temperature, steam pressure, and blade design influence the efficiency of the turbine's energy conversion.
Generator Design: Using cutting-edge materials, cooling mechanisms, and precise engineering, modern generators are made to reduce losses. By lowering the quantity of mechanical energy lost as heat, high-efficiency generators raise the total power output.
Operational Conditions: A turbine generator's efficiency is also affected by its operating environment, which includes temperature, humidity, and load demand. Sustaining optimal performance requires regular maintenance and observation.
Case Studies and Real-world Applications
Applications of steam turbines and generators in the real world show how adaptable and effective these technologies are across a range of sectors. This section presents case studies of steam turbines in contemporary power plants and performance comparisons of various turbine technologies.
Examples of Steam Turbines in Modern Power Plants
Case studies show the importance of steam turbines in power generation and offer insights into their real-world applications:
Coal-fired Power Plant: To optimize energy extraction from steam, a sizable coal-fired power plant combines high-, intermediate-, and low-pressure steam turbines. The plant has a high overall efficiency because it uses efficient turbines and cutting-edge steam cycle technologies.
Nuclear Power Plant: The thermal energy from atomic fission is transformed into mechanical energy in a nuclear power plant using steam turbines. Numerous turbine stages and reheating procedures recover as much energy as possible from the steam, helping the plant provide base-load power.
Biomass Power Plant: A biomass power plant uses wood chips and a 50kW steam turbine to produce electricity. Thanks to the turbine's connection with wood gasification technology, the plant can run sustainably by lowering its carbon footprint and dependency on fossil fuels.
Comparative Analysis of Different Turbine Technologies
The advantages and disadvantages of various strategies are revealed by comparing different turbine technologies:
Impulse vs. Reaction Turbines: The Curtis stage turbine and other impulse turbines are effective at high-pressure stages. Still, reaction turbines work best at lower-pressure stages when steam expands gradually. The power plant's particular needs will determine which of these technologies is best.
Multi-stage vs. Single-stage Turbines: Multi-stage turbines draw more energy from the steam than single-stage turbines, which are simpler and less costly. Efficiency is crucial in large-scale power generation, where multi-stage turbines are commonly employed.
Conventional vs. Renewable Fuel Turbines: While steam turbines that run on renewable fuels, like geothermal or biomass, have environmental advantages, they may also need more sophisticated fuel handling and processing systems than conventional fuel turbines.
Future Trends and Innovations in Turbine Technology
Emerging trends and technologies that aim to increase efficiency, lessen environmental impact, and broaden the spectrum of uses will affect turbine technology in the future. This section examines these trends and their effects on the electricity generation sector.
Emerging Technologies in Turbine Design
The following cutting-edge technologies have the potential to transform turbine design completely:
Supercritical CO2 Turbines: Rather than using steam as a working fluid, these turbines employ supercritical carbon dioxide. Compared to conventional steam turbines, they are more efficient and smaller, which makes them perfect for tiny power generation systems.
Advanced Blades Materials: To increase turbine longevity and efficiency, new materials, such as ceramic composites and high-temperature alloys, are being researched to handle higher temperatures and strains.
Additive Manufacturing(3D Printing): Additive manufacturing lowers production costs and improves performance by producing intricate turbine components with exact tolerances and optimal designs.
Sustainability and Environmental Considerations
Turbine technology is developing to meet these issues as environmental concerns gain importance:
Carbon Capture Integration: To lower greenhouse gas emissions, carbon capture and storage (CCS) systems are being integrated with steam turbines in fossil fuel power plants. This method removes carbon dioxide from flue gases before they are released into the atmosphere.
Hybrid Energy Systems: To create a more reliable and sustainable power source, hybrid systems that integrate steam turbines with renewable energy sources like solar thermal or wind power are being developed.
Waste Heat Recovery: Advances in waste heat recovery technology are making it possible to use thermal energy generated in industrial processes more effectively, which lowers emissions and overall energy consumption.
Conclusion
To sum up, steam turbines and associated technologies are still essential to power generation since they provide a dependable and effective way to generate electricity. Over decades of usage, these devices have demonstrated their adaptability and efficiency in a variety of settings, including small-scale, off-grid applications and large-scale power plants.
Thanks to developments in turbine design, control systems, and materials, steam turbines are becoming more efficient, eco-friendly, and flexible enough to use a variety of energy sources, including renewable fuels like biomass and wood gas.
Steam turbines will continue to be at the forefront of power-generating technology as the world shifts to a more sustainable future. They will combine with new developments and offer a reliable base for the global energy infrastructure.
This book provides a thorough review of the main ideas, innovations, and developments in engines, steam turbines, and renewable energy sources. By delving deeper into these subjects, readers can learn more about these systems' functions, uses, and significance in contemporary power generation.